Upper mantle seismic anisotropy around La Réunion hotspot and its surrounding mid-ocean ridges
Texte intégral
Figure
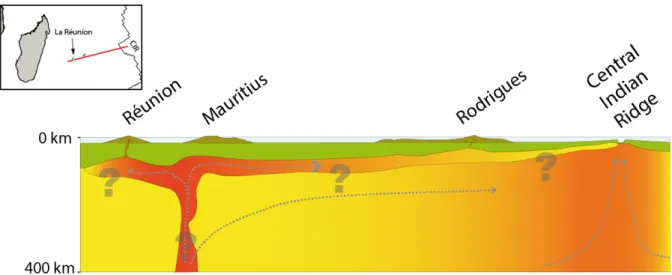
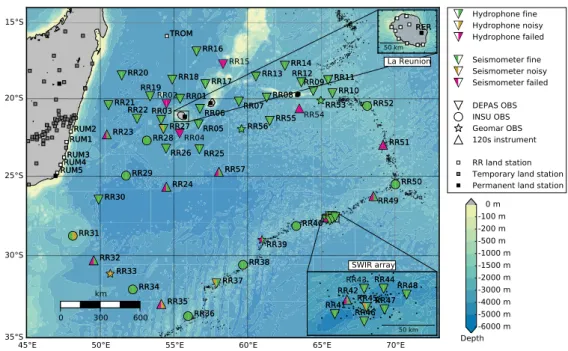
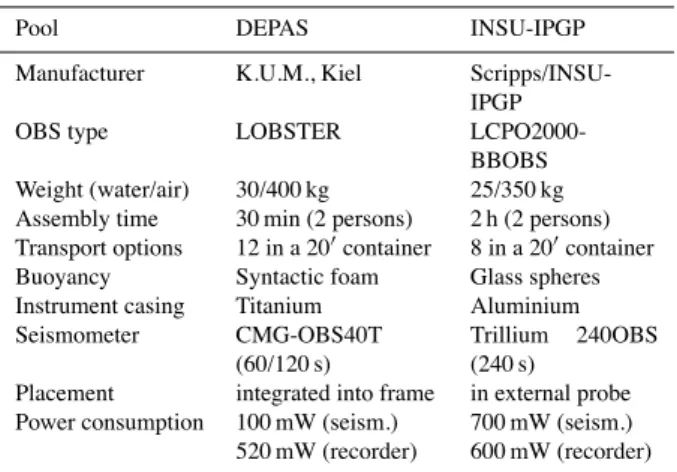

Documents relatifs
standardized steady state heavy-duty diesel engine emission testing.. Duty Cycle for nonroad applications. This and heavier loading conditions than. )iesel Duty
Jusqu’à maintenant, nous n’avons pas exploré systématiquement cette question, mais il est certain que les configurations d’orchestre électronique que nous avons mises
For power amplifier (PA) systems, digital predistortion has been a popular way to enhance the linearity of the system without sacrificing power efficiency by power
With underprivileged children in mind, the interface design was kept purposefully sim- ple and direct, so that the most novice computer user would have little trouble
The primary goal of this project was to develop a viable business model that would create tangible value to Transformer Asset Managers, enable MR to capture some of this value
The experience of watching Train of Thought v2.0 is one of exploration. The viewer sits back and follows the main narrative threads created by the system. The story
Figure 3. Perte de masse et variation de la surface contaminée des différents composites, du point d’équilibre : 23 ◦ C, 50 %HR à 30 ◦ C, 90 %HR) corrélées aux différentes
The cyclostationary mapping of supply and/or device noise from various PLL components into noise injected in the PLL loop can be described by the “Impulse Sensitivity Function”