Estimating the absolute degree of aggregation:
Texte intégral
Figure
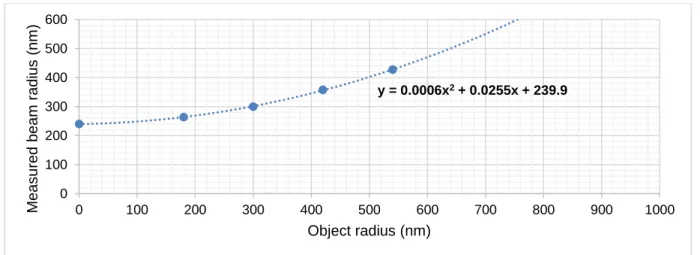
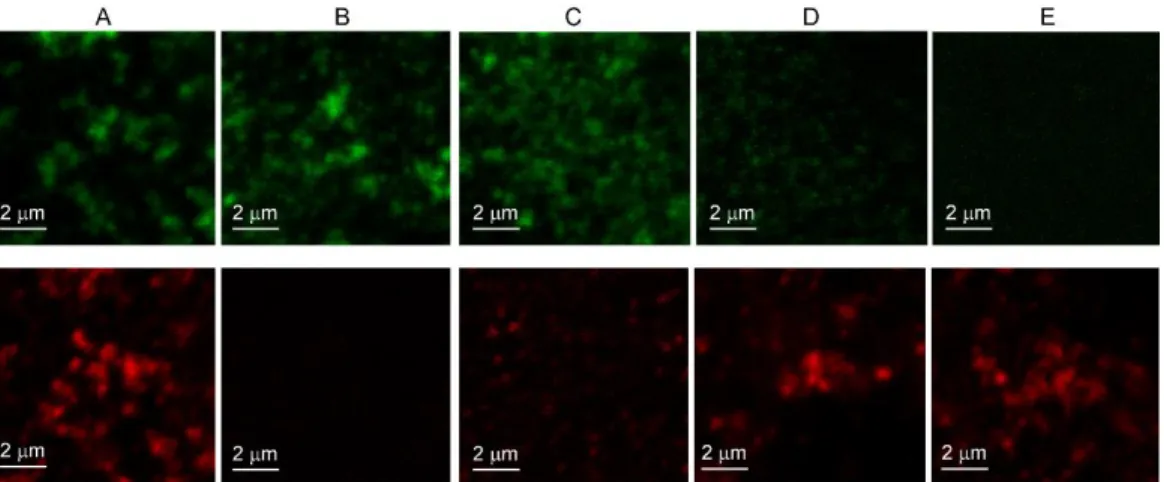

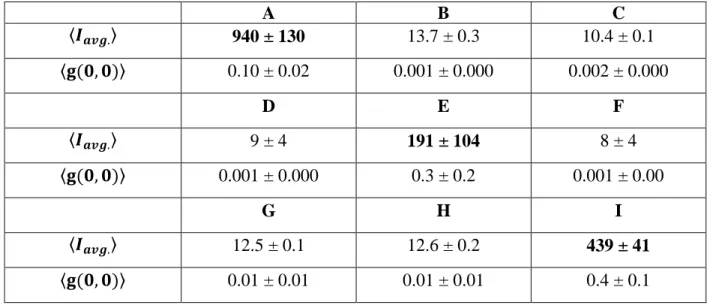
Documents relatifs
[r]
In this section we first recall the concepts of power and interaction indexes in- troduced in cooperative game theory and how the Banzhaf index can be obtained from the solution of
In the S3 blue phase this great circle corresponds to a director field line.. This corresponds to the--geometrical
As indicated in section 2, search- ing non singular director field (of nematic or blue phases) is equivalent to finding non singular
Relative concentrations of all quantified metabolites for all NMR spectra shown as average values and standard deviations for treatments and CO 2 concentrations.. Red bars
Caporaso JG, Lauber CL, Walters WA, Berg-Lyons D, Lozupone CA, Turnbaugh PJ, Fierer N, Knight R, 2011.. Global patterns of 16S rRNA diversity at a depth of millions of sequences
Degree of freedom (df), AICc, difference between AICc of each model and the minimum AICc score of the OLS models (ΔAICc) and Akaike weight (ω) of the 8 OLS models fitted from the
We propose a new approach for the generation of synthetic but visually realistic time series of cardiac images based on an electromechanical model of the heart and real clinical