Approximately half of the erythroblasts in maternal blood are of fetal origin
Texte intégral
(2) Fetal erythroblasts in maternal blood the validation of the single cell PCR results, in addition to the fetal karyotype obtained by classical cytogenetic methods. The blood samples were processed within 24 h, using the enrichment procedure we have recently described (Troeger et al., 1999). In brief, the mononucleated cells were separated with a single density gradient using Ficoll-Paque-1119® (Sigma, St Louis, MO, USA). The ring containing mononuclear cells was removed with a wide-gauge needle and washed twice with phosphate-buffered saline (PBS). The cells were resuspended in PBS containing 1% bovine serum albumin (BSA) (Sigma), incubated with anti-GPA microbeads (Miltenyi Biotec, Bergisch Gladbach, Germany) and separated using miniMACS separation columns according to the manufacturer‘s instructions. Cytospin preparations of the recovered cells were placed onto glass slides (Shandon, Frankfurt, Germany) and differentially stained with May–Gru¨ nwald–Giemsa stain (Sigma). The percentage of erythroblasts was enumerated in at least 200 nucleated cells on a cytospin preparation. The number of recovered nucleated cells was determined in a haemocytometer. The yield of erythroblasts was then calculated by the number of recovered nucleated cells times the percentage of erythroblasts. Visual detection, manipulation and digestion of erythroblasts Erythroblasts were recognized visually using an inverse microscope at ⫻400 magnification (Axiovert 100; Zeiss, Jena, Germany). Only those cells that met the following criteria were considered for micromanipulation: low nucleus-to-cytoplasm ratio, dense and small nucleus, orthochromatic and not granular cytoplasm. Erythroblasts were detached individually from the glass slides using an extended microcapillary (Drummond Scientific, Broomall, PA, USA) and a micromanipulator (Zeiss). Each erythroblast was transferred to a separate PCR tube containing 5 µl of 400 ng/µl Proteinase K and 17 µmol/l sodium dodecyl sulphate (SDS). The solution was overlaid with mineral oil and incubated at 50°C for 1 h, followed by 99°C for 30 min to extract DNA and degrade protein. Specific amplification of the β-globin, rhesus D and SRY genes A multiplex PCR was performed to amplify the β-globin, rhesus D and SRY genes simultaneously. The PCR for the β-globin gene was performed as a semi-nested PCR reaction using primers of the following sequences: external 5⬘ TCC TGA GGA GAA GTC TGC CG 3⬘, external and internal 5⬘ ACA GCA TCA GGA GTG GAC AG 3⬘, and internal 5⬘ GTG AAC GTG GAT GAA GTT GG 3⬘. The amplification of the SRY locus was done in a fully nested manner using the following pairs of primers: external 5⬘ GTG TCC TCT CGT TTT GTG AC 3⬘ and 5⬘ GAA TCA TCG CTG TTG AAT AC 3⬘, and internal 5⬘ TGG CGA TTA AGT CAA ATT CGA 3⬘ and 5⬘ CTA GTA CCC TGA CAA TGT ATT C 3⬘ respectively. In parallel the rhesus D gene was amplified with three primers by semi-nested PCR: external 5⬘ AGG GGT GTT GTA ACC GAG T 3⬘, external and internal 5⬘ CCA CAT GCC ATT GCC GGC T 3⬘, and internal 5⬘ CCC CAC AGC TCC ATC ATG 3⬘. A total of 45 µl of PCR mix containing 25 pmol of each external primer pair, 1.5 mmol/l MgCl2, 300 nmol/l dNTPs, and 2 IU Taq Polymerase (Promega, Madison, WI, USA) was added to each reaction. Amplification was carried out following a hot start at 95°C for 5 min with 30 subsequent cycles of 95°C for 30 s, 55°C for 30 s and 72°C for 60 s. 1 µl aliquots were removed from the external PCR reaction tubes and placed in 20 µl PCR mix containing 10 pmol of either one of the internal primer pairs, 1.5 mmol/l MgCl2, 300 nmol/l dNTPs, and 2 IU Taq Polymerase. The amplification was performed as above. PCR products were analysed on a 2% agarose gel and visualized using the Geldoc®. Figure 1. Multiplex polymerase chain reaction (PCR) analysis of β-globin, rhesus D and SRY loci on single cells. The PCR products are indicated by the following key: S ⫽ 100 bp DNA molecular weight marker; G ⫽ β-globin PCR product; Y ⫽ SRY PCR product; R ⫽ rhesus D PCR product. The first cell is, hence, maternal as no amplification occurred for the rhesus D and SRY loci. The second cell is from a male fetus as it is positive for all three loci, whereas the third cell is from a female rhesus D positive fetus.. analysis system (Molecular Analysist 1.4; Bio-Rad Laboratories, Hercules, CA, USA). Specific amplification of the β-globin gene, SRY locus and rhesus D gene resulted in 240, 133 and 118 bp products respectively (Figure 1). If only the β-globin gene was detected the cell was considered to be of maternal origin. If the single cell PCR revealed the same result as the fetal DNA obtained by the invasive procedures, the cell was considered as being of fetal origin. Fetal DNA obtained from cultured chorionic villus cells or amniocytes were used for confirmation of the fetal genotype. Precautions against contamination As the single cell PCR is a very sensitive method, strict precautions had been taken to avoid any contamination and to verify the results. The enrichment procedures were carried out under a laminar flow hood; each case being treated individually. Cytospins were stored in separate boxes. The micromanipulation was done in a separate room. Each cell was picked with an individual glass microcapillary, which was discarded after the procedure. PCR was performed under a separate laminar flow hood, and PCR products were analysed in a further separate room. For all procedures disposable gloves and aerosol resistant filter tips were used. Additionally all pipettes were exposed to UV radiation prior to the experiments. Negative controls (picked erythrocyte and water blanks) were included and a positive control (human male rhesus D positive DNA) was run in parallel with each case examined.. Results Enrichment procedures for erythroblasts were carried out on 19 blood samples obtained from rhesus-negative pregnant women. We were able to detect erythroblasts in 14 of these 19 cases. The number of fetal erythroblasts obtained differed in each case (see Table I). On average 1385 erythroblasts could be enriched from 15 ml of peripheral blood, ranging from 0 to 5200. In the 14 cases with erythroblasts a mean of nine cells per case were micromanipulated (range 1–33). In all, 128 erythroblasts were analysed. Specific amplification was noted in 54 of these, thereby indicating the presence of a cell and a successful PCR reaction. Of these 54 amplifications 27 were determined to be of fetal origin by either the presence of the rhesus D and/or the SRY locus. Assuming that cell loss during micromanipulation and lack of complete amplification 1163.
(3) C.Troeger et al.. Table I. Summary of the cases examined indicating gestational age, fetal genotype, number of cells manipulated and correctly examined Case. Gestation weeks ⫹ days). Gravida. Maternal blood group. Fetal rhesus type. Fetal sex. No. of cells picked. No. of successful PCR amplifications. No. of fetal cells. 1 2 3 4 5 6 7 8 9 10 11 12 13 14 15 16 17 18 19 Σ. 14⫹4 14⫹0 11⫹2 15⫹0 15⫹5 14⫹3 12⫹1 17⫹6 16⫹0 14⫹6 14⫹5 17⫹3 14⫹0 11⫹0 16⫹4 12⫹0 12⫹2 14⫹1 17⫹2. 2 2 4 2 1 1 1 2 1 3 2 4 1 1 1 2 2 3 2. Ad Ad Bd 0d Bd Ad Ad Bd Ad 0d 0d 0d 0d 0d Ad Bd 0d 0d Ad. d D d d D d D D d D D d D d D D D d D. m f m m m m f m m f f m m m f f m m f. 5 22 10 2 11 9 0 33 0 10 0 4 0 0 3 4 10 1 4 128. 5 12 0 1 6 6 0 10 0 4 0 1 0 0 1 1 6 0 1 54. 2 2 0 1 3 4 0 6 0 2 0 0 0 0 1 1 4 0 1 27. is random, these results imply that on average half of the enriched erythroblasts are of fetal origin.. Discussion The enrichment of fetal cells from the peripheral blood of pregnant women and their subsequent analysis for genetic disorders represents a challenge as they are very rare in frequency (Hamada et al., 1993; Bianchi et al., 1997). Most groups have selected the fetal erythroblast as their target cell type (Bianchi et al., 1990; Price et al., 1991; Holzgreve et al., 1992). Unlike lymphocytes, the fetal erythroblast has a short life span of ~90 days, which makes it unlikely that a false diagnosis results from fetal cells which have persisted from a previous pregnancy. A further advantage is that erythroblasts express intracellular proteins, such as fetal haemoglobin and several surface antigens, such as the transferrin receptor, blood type antigens and possible erythroid specific antigens such as HAE9 or the erythropoietin receptor, which may be useful for their enrichment and detection (Bianchi et al., 1993; Valerio et al., 1997; Oosterwijk et al., 1998; Troeger et al., 1999). A major problem, however, is that the erythroblasts present in the peripheral blood of pregnant women are of both maternal and fetal origin (Ganshirt et al., 1994; Slunga Tallberg et al., 1995; Holzgreve et al., 1998), and that no reliable way exists to distinguish between the two groups, nor is there any clear indication as to what fraction is fetal. In our approach to address this question, we used the rhesus D and the SRY genes to differentiate between both the maternal and fetal genome, by taking advantage of their absence in rhesus D negative pregnant women. As both these markers have already been analysed at the single cell level for preimplantation genetic diagnosis (Chong et al., 1993; Van den Veyver et al., 1995), their choice should facilitate a simple screening of numerous single cells. In addition, as both genes are present as single copies in the fetal genome and are absent in the 1164. maternal genome, single cell analysis should be easier to accomplish than by using heterozygous loci, such as microsatellites, where allele drop-out can lead to erroneous results (El-Hashemite and Delhanty, 1997; Garvin et al., 1998; Hahn et al., 1998b). It is interesting to note that, even though we have taken considerable steps to improve our enrichment procedure, erythroblasts were only detected in 14 out of the 19 cases examined (74%) and that a significant variation in the numbers recovered was noted (0–5200). These data are in accordance with our own previous observations (Ga¨ nshirt et al., 1994, 1998; Holzgreve et al., 1998) and those of other groups, where erythroblasts were recovered with similar frequencies even though different enrichment procedures had been used (Garcia Lloret et al., 1994; Sekizawa et al., 1996; von Eggeling et al., 1997; Valerio et al., 1997; Wachtel et al., 1998). In our PCR analysis, an informative result was obtained in 54 out of the 128 cells examined. This low degree of amplification efficiency can probably to some extent be related to cell loss during the micromanipulation, since the final transfer of the picked cell to the PCR tube is not under visual control. Several researchers have resorted to the procedure of cell pooling in order to overcome this deficiency (Cheung et al., 1996; Chan et al., 1998). However, this was not an option in our study as we needed to examine individual cells in order to determine what fraction of the enriched erythroblasts is fetal. It is possible that cell loss might be circumvented by laser-assisted micromanipulation and automatic transfer directly into the PCR tube (Schu¨ tze and Clement-Sengewald, 1994). Additionally, some template degradation may have occurred during the course of the enrichment and staining procedures (Reading et al., 1995; Cheung et al., 1996). Another possible explanation for the low amplification efficiency is that it depends to some extent on the cell type used, which may be particularly relevant for mature erythroblasts, as they are close to terminal.
(4) Fetal erythroblasts in maternal blood. differentiation and nucleus extrusion, and may hence, exhibit an increased degree of DNA damage. Despite these problems, we were nevertheless able to correctly discern fetal genotype for the two fetal (SRY and rhesus D) loci in all instances where single erythroblasts were examined. Furthermore, our results show that ~50% of the erythroblasts examined (27 out of 54) were of fetal origin by the presence of either one or both of the SRY or rhesus D loci. Although we were surprised by this high value, it concurs well with some other studies where erythroblasts were either examined by single cell PCR or fluorescence in-situ hybridization (FISH), where 30–50% of the erythroblasts were also shown to be of fetal origin (Sekizawa et al., 1996; von Eggeling et al., 1997; Oosterwijk et al., 1998; Wachtel et al., 1998). A caveat of these studies was that they either used only fetal sex or highly polymorphic nucleotide repeats for fetal identification, which in combination with the error prone procedures for whole genome amplification (Hahn et al., 1998b) that they generally used, may have introduced artefacts. Our systematic approach by the simultaneous analysis of two clearly defined fetal loci more precisely indicates the true extent of what fraction of erythroblasts in the maternal circulation is fetal. A point which still remains to be resolved is to what extent this erythroblast ratio of fetal to maternal holds true for all pregnancies. For instance, increased numbers of fetal cells have been reported for pregnancies with aneuploid fetuses (Bianchi et al., 1997), and we have observed significant elevations in erythroblast numbers in pregnancies affected by pre-eclampsia (Ga¨ nshirt et al., 1998; Holzgreve et al., 1998). In the latter instance, our results obtained by the use of FISH have indicated that ~20% of the erythroblasts in the maternal periphery are fetal (Holzgreve et al., 1998). By using a similar approach to the one we have described here, one should be able to address these issues very accurately.. Acknowledgements This work has been supported by the Swiss National Science Foundation (3200–047112.96), the National Institute of Health (Contract Number N01-HD-4–3202) and the German Research Foundation (Tr 452/1–1).. References Bianchi, D.W., Flint.A.F., Pizzimneti, M.F. et al. (1990) Isolation of fetal DNA from nucleated erythrocytes in maternal blood. Proc. Natl. Acad. Sci. USA, 87, 3279–3283. Bianchi, D.W., Zickwolf, G.K., Yih, M.C. et al. (1993) Erythroid-specific antibodies enhance detection of fetal nucleated erythrocytes in maternal blood. Prenat. Diagn., 13, 293–300. Bianchi, D.W., Williams, J.M., Sullivan, L.M. et al. (1997) PCR quantitation of fetal cells in maternal blood in normal and aneuploid pregnancies. Am. J. Hum. Genet., 61, 822–829. Chan, V., Lau, K., Yip, B. et al. (1998) Diagnosis of spinal muscular atrophy from fetal normoblasts in maternal blood. Lancet, 352, 1196–1198. Cheung, M.C., Goldberg, J.D. and Kan, Y.W. (1996) Prenatal diagnosis of sickle cell anaemia and thalassaemia by analysis of fetal cells in maternal blood. Nature Genet., 14, 264–268. Chong, S.S., Kristjansson, K., Cota, J. et al. (1993) Preimplantation prevention of X-linked disease: reliable and rapid sex determination of single human cells by restriction analysis of simultaneously amplified ZFX and ZFY sequences. Hum. Mol. Genet., 2, 1187–1191.. El-Hashemite, N. and Delhanty, J.D. (1997) A technique for eliminating allele specific amplification failure during DNA amplification of heterozygous cells for preimplantation diagnosis. Mol. Hum. Reprod., 3, 975–978. Ga¨ nshirt, D., Garritsen, H., Miny, P. and Holzgreve, W. (1994) Fetal cells in maternal circulation throughout gestation. Lancet, 343, 1038–1039. Ga¨ nshirt, D., Smeets, F.W., Dohr, A., et al. (1998) Enrichment of fetal nucleated red blood cells from the maternal circulation for prenatal diagnosis: experiences with triple density gradient and MACS based on more than 600 cases. Fetal Diagn. Ther., 13, 276–286. Garcia Lloret, M.I., Morrish, D.W., Wegmann, T.G. et al. (1994) Demonstration of functional cytokine-placental interactions: CSF-1 and GM-CSF stimulate human cytotrophoblast differentiation and peptide hormone secretion. Exp. Cell Res., 214, 46–54. Garvin, A.M., Holzgreve, W. and Hahn, S. (1998) Highly accurate analysis of heterozygous loci by single cell PCR. Nucleic Acids Res., 26, 3468–3472. Hahn, S., Sant, R. and Holzgreve, W. (1998a) Fetal cells in maternal blood: current and future perspectives. Mol. Hum. Reprod., 4, 515–521. Hahn, S., Garvin, A., Di Naro, E. and Holzgreve, W. (1998b) Allele drop out can occur in alleles differing by a single nucleotide and is not alleviated by preamplification nor minor template increments. Genetic Testing, 2, 351–355. Hamada, H., Arinami, T., Kubo, T. et al. (1993) Fetal nucleated cells in maternal peripheral blood: frequency and relationship to gestational age. Hum. Genet., 91, 427–432. Holzgreve, W., Garritsen, H.S. and Ganshirt Ahlert, D. (1992) Fetal cells in the maternal circulation. J. Reprod. Med., 37, 410–418. Holzgreve, W., Ghezzi, F., Di Naro, E. et al. (1998) Disturbed Fetomaternal cell traffic in preeclampsia. Obstet. Gynecol., 91, 669–672. Oosterwijk, J.C., Mesker, W.E., Ouwerkerk-van Velzen, M.C. et al. (1998) Fetal cell detection in maternal blood: a study in 236 samples using erythroblast morphology, DAB and HbF staining, and FISH analysis. Cytometry, 32, 178–185. Price, J.O., Elias, S., Wachtel, S.S., et al. (1991) Prenatal diagnosis with fetal cells isolated from maternal blood by multiparameter flow cytometry. Am. J. Obstet. Gynecol., 165, 1731–1737. Reading, J.P., Huffman, J.L., Wu, J.C., et al. (1995) Nucleated erythrocytes in maternal blood: quantity and quality of fetal cells in enriched populations. Hum. Reprod., 10, 2510–2515. Schu¨ tze, K. and Clement-Sengewald, A. (1994) Catch and move – cut or fuse. Nature, 368, 667–669. Sekizawa, A., Kimura, T., Sasaki, M. et al. (1996) Prenatal diagnosis of Duchenne muscular dystrophy using a single fetal nucleated erythrocyte in maternal blood. Neurology, 46, 1350–1353. Slunga Tallberg, A., el Rifai, W., Keinanen, M. et al. (1995) Maternal origin of nucleated erythrocytes in peripheral venous blood of pregnant women. Hum. Genet., 96, 53–57. Steele, C.D., Wapner, R.J., Smith, J.B. et al. (1996) Prenatal diagnosis using fetal cells isolated from maternal peripheral blood: a review. Clin. Obstet. Gynecol., 39, 801–813. Troeger, C., Holzgreve, W. and Hahn, S. (1999) A comparison of different density gradients and antibodies for enrichment of fetal erythroblasts by MACS. Prenat. Diagn., 19, 521–526. Valerio, D., Aiello, R. and Altieri, V. (1997) Isolation of fetal erythroid cells from maternal blood based on expression of erythropoietin receptors. Mol. Hum. Reprod., 3, 451–455. Van den Veyver, I.B., Chong, S.S., Cota, J. et al. (1995) Single-cell analysis of the RhD blood type for use in preimplantation diagnosis in the prevention of severe hemolytic disease of the newborn. Am. J. Obstet. Gynecol., 172, 533–540. von Eggeling, F., Michel, S., Gu¨ nther, M. et al. (1997) Determination of the origin of single nucleated cells in maternal circulation by means of random PCR and a set of length polymorphisms. Hum. Genet., 99, 266–270. Wachtel, S.S., Sammons, D., Twitty, G. et al. (1998) Charge flow separation: quantification of nucleated red blood cells in maternal blood during pregnancy. Prenat. Diagn., 18, 455–463. Received on June 2, 1999; accepted on September 9, 1999. 1165.
(5)
Figure
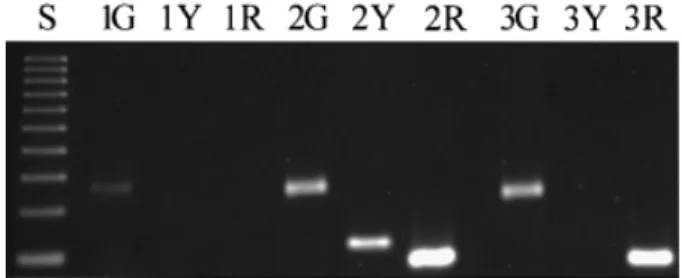

Documents relatifs
Effect of selenium supplementation on blood status and milk, urine and fecal excretion in pregnant and lactating camel. Chronic selenosis in
Qui relie les deux pôles qu’on appelle cosmiques Sont au nombre de trois pour atteindre la cible Qui est la position du grand lotus mythique L’adepte recherchant les
Die Frage stellt sich also in solchen Ringen in einer etwas anderen Form, n¨amlich ob derartige Faktorzerlegungen in unzerlegbare Elemente (bis auf Einheiten) eindeutig
ologies anchored in an earlier modernist era of empirical reasoning.
The specific objectives of the present study were to identify: 1) gilt level factors measured before inoculation that were associated with fetal mortality rate; 2) gilt and fetal
Table 2: Mean absolute 103 nmol.h/L and relative % maternal blood exposure to atrazine, propazine, simazine ATZ, PRO and SIM and their respective metabolites DEA, DIA and DACT at
Our work shows modification to seven urinary metabolites between obese, overweight, and normal-weight pregnant women, including hippurate, phenylalanine, lactate, creatine,
Our results showed that maternal and fetal exposure to BPA is associated with disruption of the thyroid function of both the mothers during pregnancy and the newborns characterized by